ST. LOUIS, MO, September 24, 2018 – For angiosperms — or flowering plants — one of the most important decisions facing them each year is when to flower. It is no trivial undertaking. To flower, they must cease vegetative growth and commit to making those energetically expensive reproductive structures that will bring about the next generation. Knowledge of this process at the cellular level is critical for understanding how plants allocate resources to produce grains, tubers, leaves, nuts and fruits that mean so much to humans and animals alike.
In a paper, Molecular basis of flowering under natural long-day conditions in Arabidopsis, published today in the journal Nature Plants, He Huang, Ph.D., research scientist, and Dmitri A. Nusinow, Ph.D., assistant member, Donald Danforth Plant Science Center, and an international team of researchers demonstrated that the gene FT — the primary driver of the transition to flowering in plants each spring — does something unexpected in Arabidopsis thaliana plants grown in natural environments, with implications for the artificial growing conditions scientists commonly use in the lab.
“This work showed us that plants are integrating many different signals together when making the critical decision of when to flower,” Dr. Nusinow said. “Subtle changes in the environment can have a big effect on how the plants will respond.”
The research team, led by University of Washington (UW) biology professor Takato Imaizumi, showed that FT has a peak of activity every morning leading up to the transition, something that scientists had not previously seen in Arabidopsis, a model plant that is widely studied for understanding the molecular details of the transition to flowering. The morning peak of FT activity causes plants to transition earlier from vegetative growth to flowering.
Prior research, which saw only an evening peak of FT gene activity, had been conducted on Arabidopsis plants grown indoors under fluorescent light. The team — which includes researchers in Switzerland, Scotland, South Korea and Japan — grew their plants outside under sunlight in Seattle because conditions at the summer solstice are similar to the standardized, artificial "long-day" growing conditions for Arabidopsis: 16 hours of light and eight hours of darkness.
The team grew non-transgenic Arabidopsis plants outdoors for five consecutive summers and compared them to plants grown indoors under artificial long-day conditions. Outdoor plants produced fewer leaves than indoor plants, indicating that the outdoor plants flowered earlier. Both outdoor and indoor plants showed evening peaks of FT gene activity, but outdoor-grown plants also showed a morning peak of FT activity. Researchers concluded that the indoor, artificial growing conditions missed key qualities of natural conditions, throwing off expression of the FT gene and the trait it governs. When active, the FT gene produces a protein that travels from the leaves to the shoot apical meristem — the niche of stem cells in the shoot that produces above-ground growth — and switches the meristem from vegetative growth to floral growth.
To identify the differences between indoor and outdoor growing conditions, the group focused on light. The fluorescent bulbs commonly used in Arabidopsis research do not emit the same wavelengths of light that sunlight does. Fluorescent bulbs, for example, generate less light from far-red wavelengths. In the outdoor growing plots, the ratio of red-wavelength light to far-red wavelength was about 1-to-1, but for fluorescent bulbs this ratio is higher than 2, which means they emit more red light than far-red. When the researchers added a far-red LED lamp to the indoor growth chambers to mimic outside light, the Arabidopsis plants then showed a morning peak of FT gene activity.
In addition, by modifying the temperatures in the indoor growth chambers to cycle daily from about 16 degrees Celsius to almost 23 C — or from 61 degrees Fahrenheit to about 73 F — the evening FT gene activity was reduced, similar to the outdoor plants.
FT has been studied in other plants, including some crop plants, which also show morning peaks of FT expression. But most commercially important plants are too large or grow too slowly for the controlled-environment studies that are required to determine the cellular and genetic details of plant traits. That is why Arabidopsis, a small, fast-growing weed from the mustard family, is widely used as a substitute model organism.
Critically, their results illuminate a path forward for plant researchers to adopt artificial growth conditions that more accurately reflect natural growing conditions.
"Arabidopsis has been studied for decades. Researchers set up their indoor growing conditions the best they could, given equipment, time and funding, and passed those conditions down to scientists they trained," said Imaizumi. "But we need to change those conditions so that what we find in the lab reflects nature more closely. If we see a change in flowering by making these minor alterations, I imagine that other traits will change as well."
“A better understanding of how the controlled environment conditions that are used in research compare to the complex reality of the outdoors will allow us to design better experiments,” Nusinow said. “This will likely push our work from simple, reductionist conditions to a richer, more complex synthesis of light and temperature that reflects the real world. This is an exciting direction for our work to be moving towards.”
Co-lead authors on the paper are former UW postdoctoral researchers Young Hun Song and Akane Kubota. Song is now an assistant professor at Ajou University and Kubota is an assistant professor at the Nara Institute of Science and Technology. Co-authors are Dmitri A. Nusinow and He Huang at the Donald Danforth Plant Science Center; Michael Kwon, Nayoung Lee, Ella Taagen, Dianne Laboy Cintrón, and Nhu Nguyen at the University of Washington; Michael Covington with Amaryllis Nucleics; Dae Yeon Hwang at Ajou University; Sarah Hodge and Andrew Millar at the University of Edinburgh; Reiko Akiyama with the University of Zurich; and Kentaro Shimizu of both the University of Zurich and Yokohama City University.
The research was funded by the U.S. National Institutes of Health, the U.S. National Science Foundation, the Rural Development Administration in South Korea, the Japan Science and Technology Agency, the Japan Society for the Promotion of Science, the Swiss National Science Foundation, UK Biotechnology and Biological Sciences Research Council, and the National Research Foundation of Korea.
About The Donald Danforth Plant Science Center
Founded in 1998, the Donald Danforth Plant Science Center is a not-for-profit research institute with a mission to improve the human condition through plant science. Research, education and outreach aim to have impact at the nexus of food security and the environment, and position the St. Louis region as a world center for plant science. The Center’s work is funded through competitive grants from many sources, including the National Institutes of Health, U.S. Department of Energy, National Science Foundation, and the Bill & Melinda Gates Foundation. Follow us on Twitter at @DanforthCenter.
Video: Seasonal Flowering
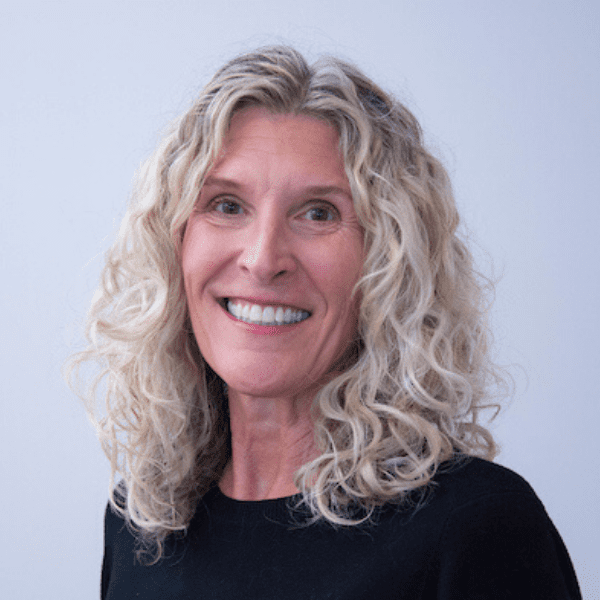